Developing a method for direct bonding single-crystal diamond membranes to a wide variety of materials.
Science: Scientists at Argonne National Laboratory and the University of Chicago directly bond single-crystal diamond membranes to a variety of materials, demonstrating multiple methods for integrating high-quality-factor nanophotonic cavities with diamond heterostructures.
Impact: This enables added versatility in design of portable devices based on spin defects in diamond, which are highly promising for quantum sensing.
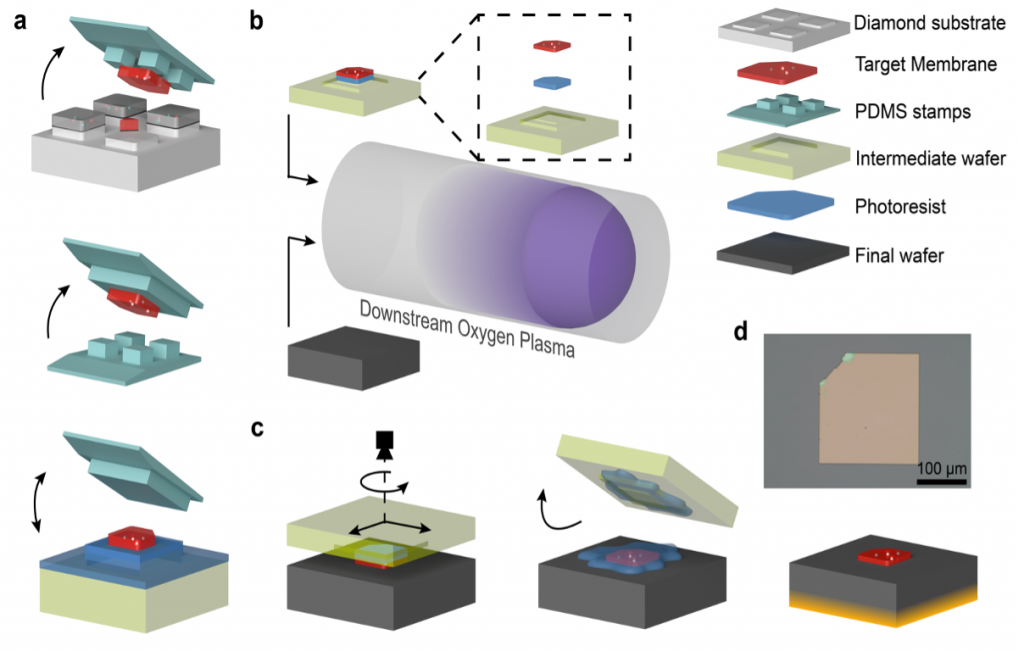
Summary: Ultrathin diamond membranes are created based on “smart cut”, overgrowth, and etching. Membranes are implanted with spin qubits and can be transferred to carrier wafers using a polydimethylsiloxane (PDMS) stamping process. The qubits’ integration with nanophotonic resonators can translate to high quality factors more than 104. The process has a high yield, with high quality interfaces between diamond and the substrate (e.g., fused silica, sapphire, LiNbO3).
Contact: Alex High, ahigh@uchicago.edu
Focus area: Quantum foundries
Institutions: Argonne National Laboratory, University of Chicago
Citation: X. Guo, M. Xie, A. Addhya, A. Linder, U. Zvi, T. D. Deshmukh, Y. Liu, I. N. Hammock, Z. Li, C. T. DeVault, A. Butcher, A. P. Esser-Kahn, D. D. Awschalom, N. Delegan, P. C. Maurer, F. J. Heremans, A. A. High. “Direct-bonded diamond membranes for heterogeneous quantum and electronic technologies.” (2023)
Funding acknowledgment: This work is primarily funded through Q-NEXT, supported by the U.S. Department of Energy, Office of Science, and National Quantum Information Science Research Centers. Growth-related efforts were supported by the U.S. Department of Energy, Office of Basic Energy Sciences, Materials Science and Engineering Division (N.D.). The membrane bonding work is supported by NSF award AM-2240399. The quantum metrology and sensing demonstration is supported by the 12 U.S. National Science Foundation (NSF) Quantum Idea Incubator for Transformational Advances in Quantum Systems (QII-TAQS) for Quantum Metrological Platform for Single-Molecule Bio-Sensing (NSF OMA-1936118), and Quantum Leap Challenge Institute (QLCICI) for Quantum Sensing in Biophysics and Bioengineering (QuBBE) (NSF OMA-2121044). This work made use of the Pritzker Nanofabrication Facility (Soft and Hybrid Nanotechnology Experimental Resource, NSF ECCS-2025633) and the Materials Research Science and Engineering Center (NSF DMR-2011854) at the University of Chicago. Work performed at the Center for Nanoscale Materials, a U.S. Department of Energy Office of Science User Facility, was supported by the U.S. DOE, Office of Basic Energy Sciences, under Contract No. DE-AC02-06CH11357. The quantum photonics work and part of the membrane bonding work is supported by the Quantum Leap Challenge Institute for Hybrid Quantum Architectures and Networks (HQAN) (NSF OMA-2016136). A. Addhya additionally acknowledges support from Kadanoff-Rice fellowship (NSF DMR-2011854). C. DeVault receives support from the CQE IBM postdoctoral fellowship training program. The authors thank Dr. David A. Czaplewski for valuable discussion and experimental guidance, Dr. Kazuhiro Kuruma, Alexander Stramma, Hope Lee and Zander Galluppi for providing materials, and Dr. Peter Duda and Dr. Yizhong Huang for nanofabrication assistance.